When we talk about nuclear, we often associate it with the production of energy. What is less well-known, however, is the importance of nuclear in everyday life. We spoke to Davide Bortot, a researcher at the Department of Energy at the Politecnico di Milano, who works on nuclear measurements and instrumentation.
What nuclear applications are there in everyday life?
One of the most common fields of application of radiation is in medicine. This sector, for example, can be divided into two areas: diagnostic imaging and radiotherapy. The first encompasses a range of non-invasive techniques used to capture images of a patient’s condition, whether critical or less so. These are exams that everyone has heard of: from simple X-rays to 3D computed tomographies (CT scans). These techniques are known as “transmission imaging”: a generator emits radiation which passes through the patient’s body and, depending on that which the radiation meets, an image is produced. This occurs due to the differences in the interactions of the radiation with the material, human tissue in this case. It is possible to create a two-dimensional image with a simple X-ray, or a three-dimensional image with a volume tomography of the organ or part of the body under examination.
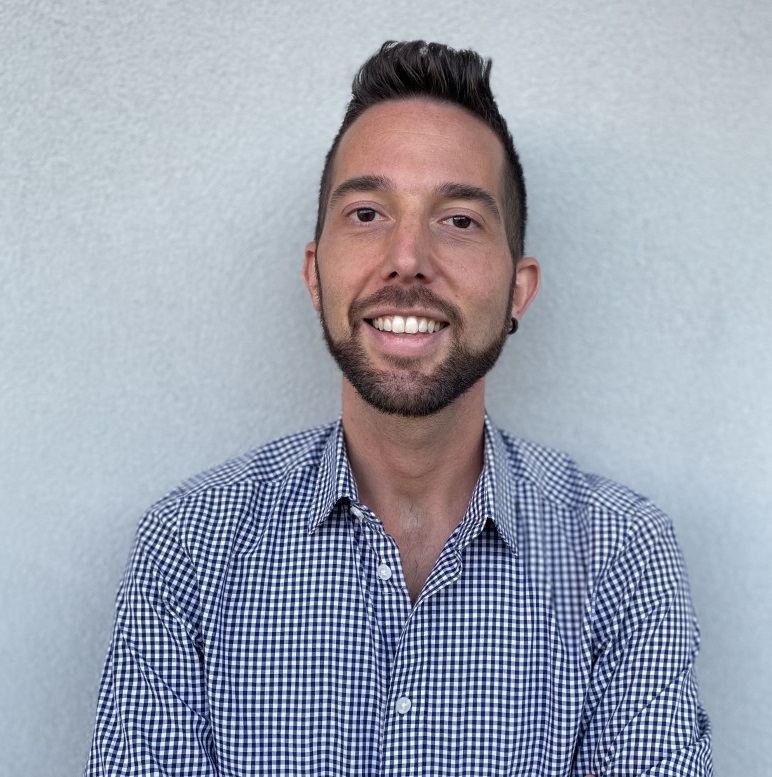
And what emission imaging techniques are there?
This is where it is the patient that becomes “radioactive”, in the sense that he or she is administered with a medication called a radiopharmaceutical, because it contains a radioactive tracer. The patient then undergoes a scan using external detectors, which measure the radiation emitted by his or her body. There are two main techniques: SPECT (Single Photon Emission Computed Tomography) and PET (Positron Emission Tomography). These techniques are very important, since they are capable of providing information regarding the function of the organ in question. Whereas transmission imaging techniques, such as CT scans, show the morphology of a patient very clearly and allow us to identify tumour-like lesions for example, on the other hand, a PET measures the function of an organ more accurately, because the transit of the radiopharmaceutical within the patient’s body is examined over space and time. Therefore, we can study the function of the organ and ascertain whether it is correct, or whether there are anomalies compared to normal function.
Are there any risks of using radiopharmaceuticals?
The patient cannot remain radioactive for long, hence the radiopharmaceuticals consist of radionuclides which have a reasonably short half-life. For this reason, they must be produced ad hoc on a daily basis by hospitals or centres which, generally speaking, house particle accelerators.
How can radiation be applied in the medical sector other than for diagnostic imaging?
The use of nuclear radiation to treat tumours is the other important field of medical application. There are essentially three techniques which are most often used in the treatment of cancer: surgery, chemotherapy and radiotherapy. So, depending on the type of tumour, the stage of the cancer and the patient’s characteristics, we can opt for one of these three or sometimes even a combination of two or three. The idea of radiotherapy is to utilise the radiation for the purpose of destroying the tumour, targeting the tumour cells, always keeping in mind that we must preserve the healthy tissue as much as possible. There is always a compromise between the amount of radiation that we impart to a certain tissue, so as to limit any complications for the healthy tissue, and to combat the tumour cells.
What are the most advanced techniques for the treatment of tumours?
Radiotherapy itself can be divided into conventional radiotherapy (based on electron and electromagnetic radiation) and hadrontherapy, that is the treatment of tumours using hadrons, which include protons, carbon ions and neutrons. Hadrontherapy is particularly suited to the treatment of radioresistant tumours, tumours that do not respond to traditional radiotherapy with X-rays or inoperable tumours. A major advantage of hadrontherapy compared to conventional treatment is the ability to exploit the fact that these types of particles, especially protons and carbon ions, have a different way of interacting with materials, including a patient’s body, and so they are able to deposit a great deal of energy when they are about to stop. This allows us to treat tumours in particularly difficult locations, operating with utmost precision and preserving the healthy tissue.
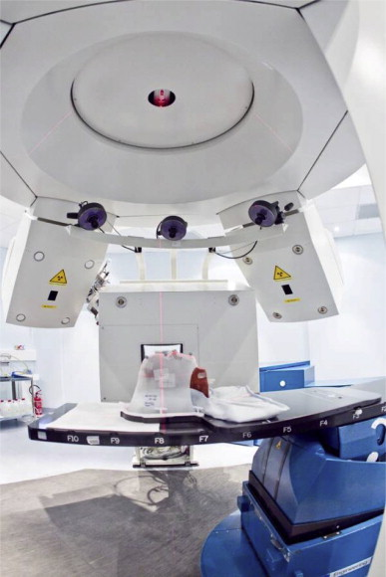
What is the future of tumour treatment?
Carbon ion treatment, which is very expensive, requires a more complex acceleration technique. As such, there are very few centres across the world. One of these is in Pavia, Italy, at CNAO, which stands for the National Center of Oncological Hadrontherapy. A particle accelerator will soon be installed at CNAO which will generate fields of neutrons for the development of an experimental radiotherapy treatment: Boron Neutron Capture Therapy, BNCT. This therapy involves the administration of a medication which is capable of transporting a non-radioactive isotope of boron, Boron-10, to within the tumour cells. The difference in metabolism of these cells compared to those of healthy tissue means that the isotope accumulates in greater quantities in the tumour cells. When the tumour is irradiated with the neutrons, the nucleus of the Boron-10 captures a neutron, triggering a reaction which produces two ionising particles which are capable of destroying the tumour cell.
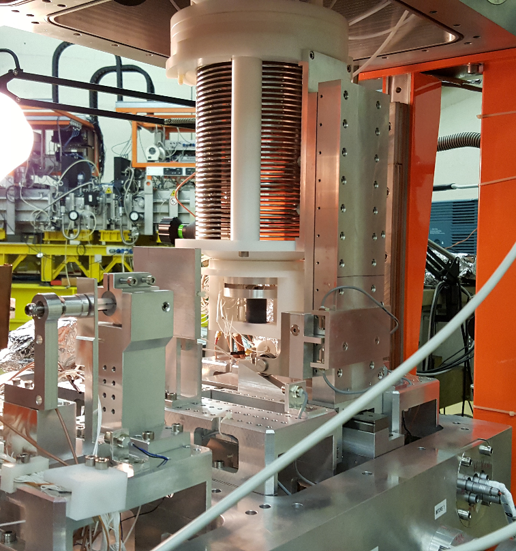
What do you do at the Politecnico?
I work in the Laboratory of Electronic and Nuclear Measurements in the Department of Energy. What we do is study and develop the innovative particle detectors that are able to distinguish the complex radiation fields, including those in the medical sector. We deal with complex and mixed fields of radiation. What we do is measure and analyse the characteristics of this type of fields, using innovative or conventional detectors depending on our objective. With regard to radiotherapy, for example, the research that I started during my doctorate relates to the so-called field of micro- and nanodosimetry. Currently, radiotherapy treatments always make reference to the scale of the dose absorbed, which is of importance when we think in macroscopic volumes. However, when we begin to get down to very small volumes, on the scale of the dimensions of the cell or the substructure of the cell (chromatin fibres or DNA, dimensions that range from one to a few microns, up to approximately 10 nanometres), the interaction of the radiation with the material is very difficult to describe. If we think in very small dimensions, the stochasticity of the interaction between the particle and the material comes into play. Therefore, the concept of the dose absorbed begins to lose its validity. The aim of microdosimetry is specifically to study the interaction of the radiation with the target and the perceivable volumes, which lead to the final damage. Conventional detectors are not suited to doing this; thus we are working on so-called microdosimeters, that is detectors which are capable of simulating the typical dimensions of a cell. And the examination of the reaction with this type of tool allows us to obtain information on the quality of the radiation beam, which is much more detailed information.
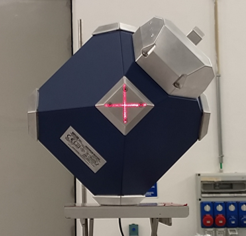
What is the added value of doing research at the Politecnico?
Being an engineer and being a researcher at the Politecnico brings a huge benefit. We work a lot with other groups of researchers. We appreciate how having engineering skills gives us the ability to succeed in developing devices. We, as researchers at the Politecnico and as engineers, are able to study the problem, but we are also able to provide a solution which is more advanced from an engineering perspective, which can lead to increase in quality, even just in the development of a new detector. Therefore, this is the added value, or rather being able to work on the parameters, working on the engineering of a product, in order to obtain a research tool and bring it ever closer to its utilisation by the final user.
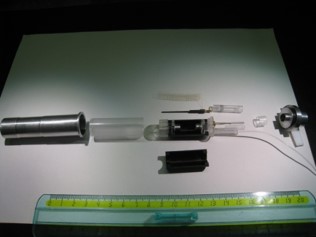